Introduction
Mendelian genetics is the bedrock of inheritance studies. It helps us understand how traits pass from one generation to the next. Think of it as the original recipe book for biology, detailing how characteristics like eye color and height are inherited. Gregor Mendel, the father of genetics, conducted meticulous experiments with pea plants. His groundbreaking discoveries laid the groundwork for modern genetics.
Mendel’s principles are more than just academic; they have real-world implications. Imagine a parent hoping to understand the risk of passing on a genetic disorder to their child. This is where probability, pedigrees, and chi-square statistics come into play. Probability helps predict the likelihood of inheriting certain traits. Pedigree charts visually map out genetic inheritance across generations, while chi-square statistics allow researchers to test hypotheses about observed genetic ratios.
Understanding how to apply statistical methods is crucial in analyzing genetic data.
These tools are vital for genetic counseling and disease prevention. For example, if a family has a history of sickle cell anemia, genetic counselors can use these methods to assess risk and provide informed options. Understanding these concepts equips families with knowledge, potentially changing lives. By utilizing Mendelian genetics, we can demystify inheritance patterns and make informed decisions about health and well-being.
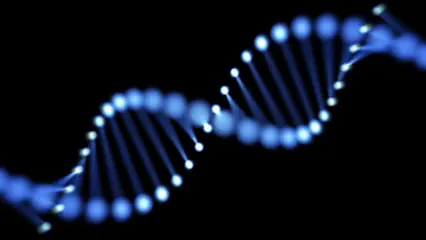
If you’re keen on diving deeper into genetics, why not check out Genetics: A Conceptual Approach by Benjamin A. Pierce? This book is like the GPS for navigating the complex world of genetics—helping you find your way through the maze of genetic theory and application!
Ready to explore the fascinating world of genetics? Buckle up, and let’s navigate through the captivating principles of Mendelian genetics, starting with its foundational concepts.
Mendel’s Laws of Inheritance
Mendel’s First Law (Law of Segregation)
Mendel’s First Law, or the Law of Segregation, is a cornerstone of genetic inheritance. It states that alleles, the different versions of a gene, separate during gamete formation. This means that each parent contributes one allele for each trait to their offspring. Imagine a game of genetic roulette! When gametes (sperm and egg) combine, offspring receive a mix of traits from both parents.
For example, if one parent has the genotype Aa (where “A” is dominant and “a” is recessive), the gametes produced could be A or a. This segregation ensures genetic diversity, as offspring can inherit different combinations of alleles, leading to a variety of traits. So, every time two individuals mate, they are essentially rolling the dice on which traits get passed down!
And if you’re curious to learn more about the science behind these laws, consider picking up The Selfish Gene by Richard Dawkins. It offers a unique perspective on how genes drive evolution and inheritance!
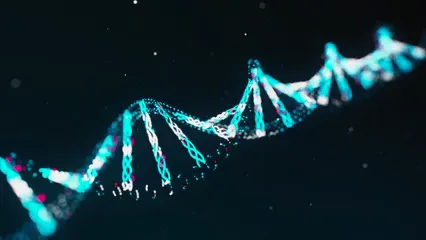
Mendel’s Second Law (Law of Independent Assortment)
Mendel’s Second Law, the Law of Independent Assortment, takes things up a notch. It posits that the inheritance of one trait does not influence the inheritance of another. Each trait segregates independently during gamete formation.
Consider this: if we track two traits, like seed shape (round vs. wrinkled) and seed color (yellow vs. green), the alleles for these traits will assort independently. That means the combination of traits in the offspring can vary widely. You could end up with round yellow seeds, round green seeds, wrinkled yellow seeds, or wrinkled green seeds! This law emphasizes the complexity and beauty of genetic inheritance, allowing for even more diversity in traits.
For those who want to get creative while learning, check out The Art of Genetics: A Coloring Book for Kids. It’s a fun way to introduce the younger generation to these concepts!
Importance of Punnett Squares
How to Use Punnett Squares
Punnett squares are a nifty tool for predicting genetic outcomes. Here’s how to construct one for both monohybrid and dihybrid crosses:
- Identify the Parent Genotypes: Determine the genetic makeup of the parents. For a monohybrid cross, let’s say one parent is homozygous dominant (AA) and the other is homozygous recessive (aa).
- Set Up the Square: Draw a two-by-two grid for a monohybrid cross. For a dihybrid cross, use a four-by-four grid.
- Fill in the Alleles: Write the alleles of one parent along the top and the other parent along the side.
- Combine the Alleles: Fill in each box of the grid by combining the alleles from the top and side.
- Analyze the Results: Count the genotypes to determine the expected ratios.
For example, if you cross AA x aa, all offspring will be Aa, showcasing the dominance of “A”.
To get a deeper understanding of these tools, grab Genetics for Dummies by Tara Rodden Robinson. It’s a straightforward guide that breaks down the complexities of genetics!
Examples
Let’s illustrate with a simple example. Consider a monohybrid cross between a plant with purple flowers (PP) and one with white flowers (pp):
– Set up the Punnett square:
| | P | P | |---|---|---| | p | Pp | Pp | | p | Pp | Pp |
From this, we see all offspring will have the genotype Pp, thus all will display purple flowers.
Now, for a dihybrid cross, let’s take a plant with round yellow seeds (RrYy) crossed with a plant with wrinkled green seeds (rryy):
| | RY | Ry | rY | ry | |---|----|----|----|----| | rY | RrYy | Rryy | rrYy | rryy | | ry | RrYy | Rryy | rrYy | rryy |
From this cross, we can predict the phenotypic ratio of the offspring. Expect to see a combination of round yellow, round green, wrinkled yellow, and wrinkled green seeds.
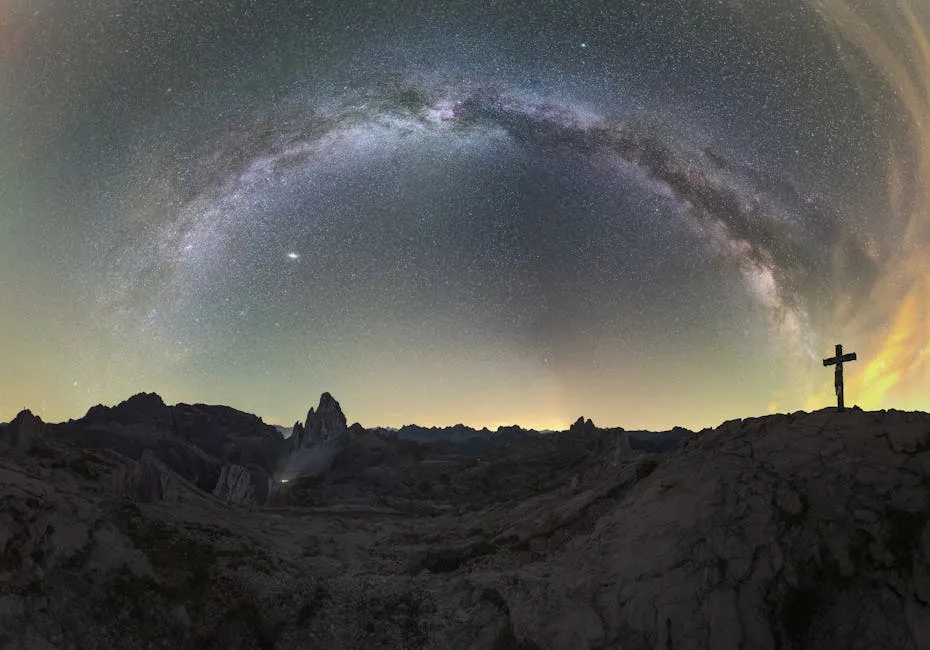
Probability in Genetics
Basic Concepts of Probability
Probability is like the crystal ball of genetics. It allows us to calculate the likelihood of inheriting specific traits. In genetics, probability helps predict the chances of offspring displaying dominant or recessive traits.
For instance, when crossing two heterozygous parents (Aa x Aa) for a single trait, the expected probability of offspring exhibiting the dominant trait is 75%. This calculation stems from the potential combinations of alleles that can arise during gamete formation.
To simplify, here’s how it works: If you toss a coin, the chance of landing on heads is 50%. Similarly, when dealing with genetic traits, you can express the chance of inheriting a dominant trait as a fraction or percentage.
Understanding these basic probability principles allows geneticists to make informed predictions about traits in offspring. The potential combinations of alleles can lead to diverse outcomes, much like a game of chance!
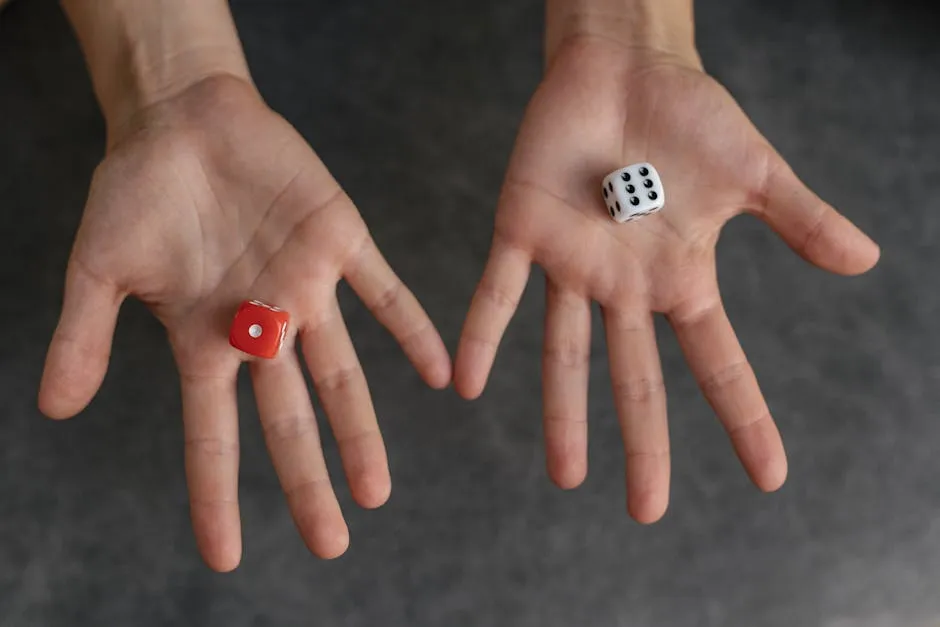
Applying Probability to Genetic Predictions
Example Scenarios
Let’s have some fun with probability! Imagine you’re a genetic wizard, mixing alleles like a cocktail. In a monohybrid cross, you might cross two heterozygous pea plants (Aa x Aa) to see the magic unfold. The expected ratio? It’s a classic 3:1! This means three out of four offspring will express the dominant trait. Picture this: if you have a garden of 100 plants, you could expect about 75 to flaunt those vibrant purple flowers, while 25 might shy away, showing off their white blooms.
Now, let’s kick it up a notch with a dihybrid cross. Cross two plants, one with round yellow seeds (RrYy) and the other with wrinkled green seeds (rryy). The expected ratio here is a dazzling 9:3:3:1 for the phenotypes! So, if you had 160 seeds from this cross, you’d expect around 90 to be round yellow, 30 round green, 30 wrinkled yellow, and 10 wrinkled green. That’s a colorful garden!
For a comprehensive guide on understanding these principles, Genetics and Genomics in Medicine by A. J. C. van der Voet would be a great addition to your library!
Real-World Applications
Probability isn’t just for plant enthusiasts; it’s a game-changer in predicting genetic disorders too! Imagine a couple wanting to know the chances of their child inheriting a genetic disorder like cystic fibrosis. By using probability, genetic counselors can estimate the likelihood of passing on specific traits.
Let’s say both parents are carriers of the cystic fibrosis gene (CF). With each pregnancy, there’s a 25% chance their child could inherit the disorder. This information empowers families to make informed decisions about family planning, medical care, and lifestyle adjustments.
Probability in genetics is also pivotal for animal breeding programs. Breeders use these calculations to select traits they desire in the next generation. Want to produce a litter of hypoallergenic puppies? Probability helps predict which pairings might yield that perfect pup.
In essence, probability provides a roadmap for understanding genetic inheritance, guiding families and breeders alike toward informed choices.
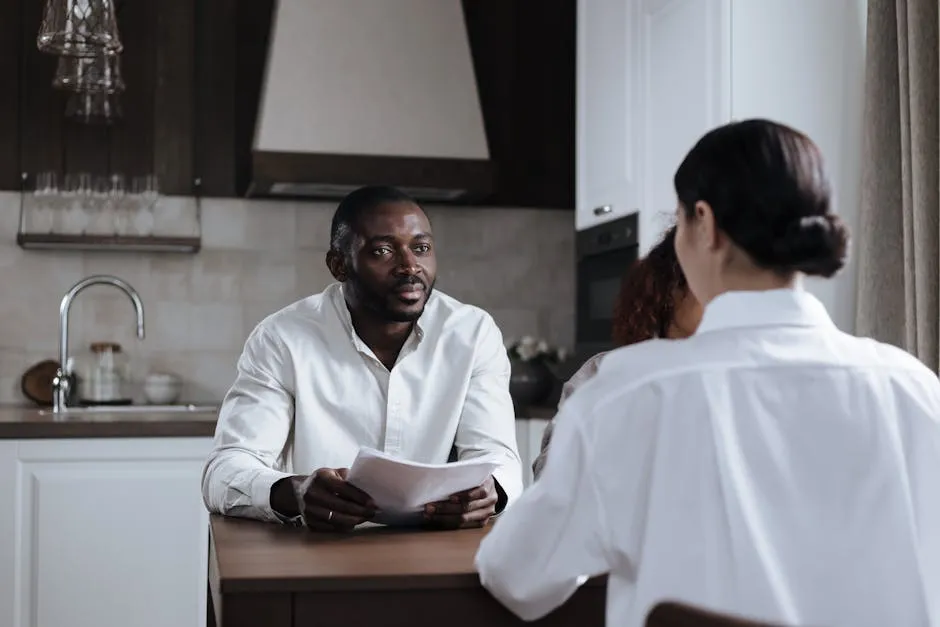
Pedigree Analysis
Understanding Pedigrees
A pedigree chart is like a family tree, but it’s got a genetic twist. It visually represents the inheritance of traits across generations. Think of it as a genetic snapshot, where you can trace traits like eye color, blood type, or genetic disorders through familial connections. Pedigree charts help geneticists and families alike understand how traits are passed down, revealing patterns of inheritance that could inform future generations.

Constructing a Pedigree
Creating a pedigree chart is easier than you think! Follow these steps to map out your genetic history:
- Start with Family Members: Gather information about your family. Who are the key players? Parents, siblings, grandparents—you’ll want to include anyone relevant to the traits you’re tracking.
- Use Standard Symbols: In pedigree charts, males are represented by squares, and females by circles. A filled symbol indicates an affected individual, while an empty one shows an unaffected person. It’s a simple and effective way to signify genetic traits.
- Connect Relationships: Draw lines to connect parents to their offspring. A horizontal line between two symbols represents a mating pair, while vertical lines lead to their children.
- Add Generational Layers: Arrange the symbols from top to bottom, with the oldest generation at the top and the youngest at the bottom. This creates a clear lineage.
- Label with Details: Include any relevant genetic information, such as genotypes or phenotypes next to the symbols. This adds depth to your chart!
Case Study: Sickle Cell Anemia
Let’s take a closer look at sickle cell anemia, a genetic disorder that affects hemoglobin in red blood cells. In a pedigree chart, you might notice a pattern: filled symbols where individuals have the disorder (SS genotype) and half-filled symbols for carriers (AS genotype).
For instance, if a couple has one child with sickle cell anemia and one without, you can track the trait back through their parents. This visual representation helps illustrate how the disorder might be passed down through generations. It’s a powerful tool for understanding not just the present, but also the potential future health of offspring.
If you’re keen on understanding more about genetic disorders, grab a copy of Sickle Cell Disease: A Guide for Patients and Families. It’s an invaluable resource for those affected!
By using pedigree analysis, families can better understand their genetic history. This knowledge is invaluable in genetic counseling, where professionals help families assess risks and make informed decisions regarding health. Pedigree charts make complex information accessible, paving the way for a healthier future.
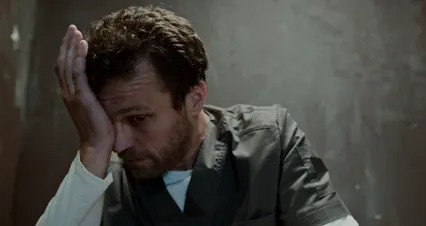
Analyzing Inheritance Patterns
Identifying Modes of Inheritance
When analyzing pedigrees, understanding the mode of inheritance is crucial. It helps us decipher whether a trait is autosomal dominant, autosomal recessive, or X-linked. Let’s break it down!
Autosomal Dominant Traits: These traits usually appear in every generation. If one parent has the trait, there’s a 50% chance their child will inherit it. Look for the classic pattern: affected individuals have at least one affected parent. For instance, if a trait like Huntington’s disease shows up in successive generations, it’s likely autosomal dominant.
Autosomal Recessive Traits: These traits can skip generations. An individual may express the trait even if neither parent shows it. Why? Both parents might be carriers. If two carrier parents have children, there’s a 25% chance the child will express the trait. Take cystic fibrosis as an example: often, unaffected parents can have affected children.
X-Linked Traits: Here’s where things get a bit more complicated. X-linked traits often affect males more than females. Why? Males have one X chromosome. If that X carries a recessive trait, voila! They express it. Females, having two X chromosomes, would need both to carry the recessive trait to express it. A prime example is hemophilia, which predominantly affects males in the family tree.
For an in-depth understanding of these concepts, consider The Gene: An Intimate History by Siddhartha Mukherjee. It’s a captivating exploration of the gene and its profound implications!
By analyzing these patterns, you can decode the mystery of inheritance and make predictions about future generations.

Chi-Square Statistics in Genetics
Introduction to Chi-Square Statistics
The chi-square test is a powerful statistical tool in genetics. It allows researchers to compare observed data with expected ratios. Why is this significant? It helps determine if the differences between observed and expected results are due to chance or if they signify something more substantial.
In genetics, we often deal with Mendelian ratios. For instance, when studying traits in pea plants, we expect a 3:1 ratio for dominant to recessive traits in monohybrid crosses. The chi-square test helps assess if our actual results align with these expectations. If not, it might signal a deviation from classic Mendelian inheritance.

Performing a Chi-Square Test
Conducting a chi-square test involves several straightforward steps. Follow along!
- Define Observed and Expected Values: Start by collecting your data. The observed values are what you find in your experiments, while expected values are what you predict based on Mendelian ratios.
- Calculate the Chi-Square Statistic: Use the formula: \[ \chi^2 = \sum \frac{(O – E)^2}{E} \] Here, \(O\) represents observed values, and \(E\) denotes expected values. This formula helps quantify the differences.
- Determine Degrees of Freedom (df): Degrees of freedom is calculated as the number of categories minus one. For example, if studying a trait with four phenotypic categories, df would be 3 (4 – 1).
- Compare the Calculated Chi-Square Value to Critical Values: Refer to a chi-square distribution table. This helps you see if your calculated value falls within an acceptable range. If it exceeds the critical value for your chosen significance level (typically 0.05), you may reject the null hypothesis—it means your data significantly deviates from the expected ratios!
By following these steps, you can assess whether your genetic crosses conform to Mendelian principles or if something unusual is at play. The chi-square test is a bridge between observation and theory in the fascinating world of genetics!
Example Calculations
Case Study: Let’s consider a classic example involving pea plants. Imagine you’re studying a monohybrid cross between a plant with a dominant purple flower color (P) and a plant with a recessive white flower color (p). If both parents are crossed (Pp x Pp), we expect a phenotypic ratio of 3:1 (three purple to one white).
Now, let’s say you observe the following results from your experiment: 75 purple and 25 white flowers. To test if these results fit the expected Mendelian ratio, you can apply the chi-square test.
Calculating:
- For purple: \(\frac{(75 – 75)^2}{75} = 0\)
- For white: \(\frac{(25 – 25)^2}{25} = 0\)
So, \(\chi^2 = 0 + 0 = 0\).
Interpreting Results: The next step is interpreting the chi-square value. With a calculated \(\chi^2\) of 0 and degrees of freedom (df) of 1 (since we have two categories, we use df = n – 1), we can compare this against a chi-square distribution table.
At a significance level of 0.05, the critical value for df = 1 is 3.84. Since 0 < 3.84, we fail to reject the null hypothesis. This means our observed flower color distribution aligns with the expected Mendelian ratio. In simpler terms, our experiment supports Mendel’s findings!
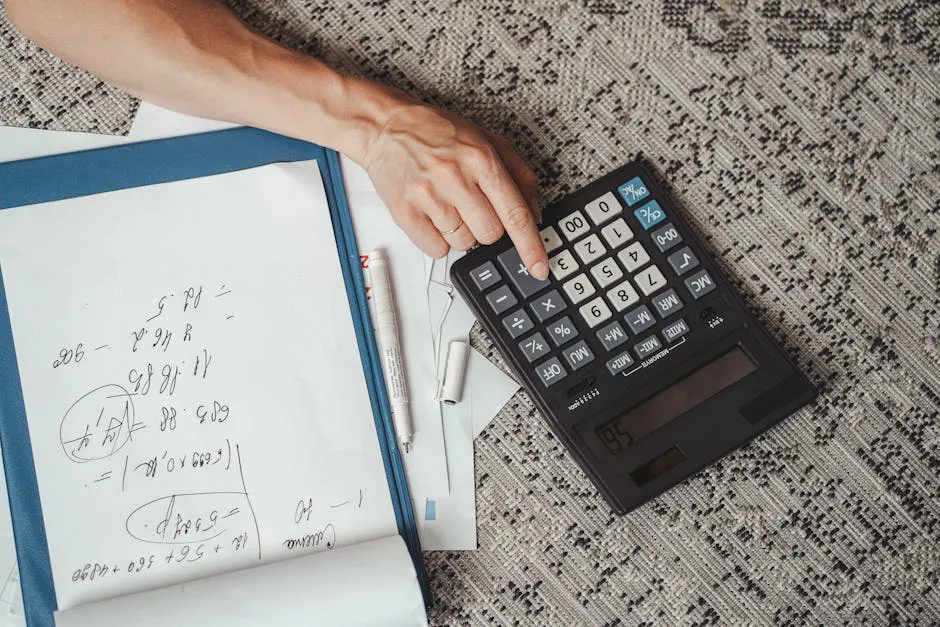
Real-World Applications of Genetics
Genetic Counseling
Understanding Mendelian genetics is crucial in genetic counseling. Parents with a family history of genetic disorders, like cystic fibrosis, seek guidance on the likelihood of passing on conditions to their children. By constructing pedigrees and applying probability, counselors can assess risks accurately. This allows families to make informed decisions about family planning and management of potential health issues.
Moreover, knowledge of inheritance patterns equips genetic counselors to provide tailored advice. If one parent is a carrier of a recessive trait, such as sickle cell anemia, understanding the probabilities aids in discussing the chances of having affected offspring. This knowledge empowers families, offering insight into their genetic landscape and informing reproductive choices.

Medical Implications
Genetic analysis isn’t just for family planning; it plays a pivotal role in diagnosing and managing inherited diseases. Take sickle cell disease, for example. By understanding the genetics behind the condition, healthcare professionals can implement early screening programs. This enables timely intervention for affected individuals, potentially improving their quality of life.
In the case of genetically linked cancers, such as BRCA mutations, awareness allows for proactive measures. Individuals with a family history can undergo genetic testing. Here, probability and pedigree analysis come into play, guiding them in making informed decisions about preventive surgeries or increased surveillance.
Additionally, genetic insights contribute to personalized medicine. Treatments tailored to an individual’s genetic makeup are becoming more common. This approach maximizes effectiveness and minimizes adverse effects. As research advances, we can expect genetic information to continue shaping the future of medicine, making it more precise and effective.
Conclusion
Recap
In this exploration of Mendelian genetics, we’ve unraveled the principles of inheritance, the roles of probability and pedigrees, and the significance of chi-square statistics. These concepts are not just theoretical; they hold real-world implications in genetic counseling and disease management.
Future Directions
Ongoing research in genetics promises to expand our understanding of complex traits and genetic disorders. As technology advances, the integration of statistical methods into genetic studies will enhance our ability to decipher intricate inheritance patterns. This opens up exciting possibilities for new discoveries and innovations in healthcare.
Call to Action
Want to dive deeper into the world of genetics? Explore resources available online, attend workshops, or even consider genetic counseling. Understanding your genetic makeup can empower you in making informed health decisions, impacting your life and future generations. Embrace the journey of discovery in genetics and its vast applications!
FAQs
What is Mendelian genetics?
Mendelian genetics is the study of how traits are inherited through generations, based on Gregor Mendel’s principles of dominance and segregation. It provides the foundation for our understanding of genetic inheritance.
How do probability and genetics relate?
Probability in genetics predicts the likelihood of inheriting specific traits. By calculating potential outcomes from genetic crosses, we can assess the chances of traits manifesting in offspring.
What are pedigree charts used for?
Pedigree charts visually represent family histories, highlighting the inheritance patterns of traits over generations. They are essential tools in genetic counseling to assess risks for hereditary conditions.
How is the chi-square test used in genetics?
The chi-square test validates genetic hypotheses by comparing observed data with expected ratios. It helps determine if the differences are due to chance or indicate significant deviations from expected inheritance patterns.
Please let us know what you think about our content by leaving a comment down below!
Thank you for reading till here 🙂
All images from Pexels
First, calculate the expected numbers:
- Purple: 75% of 100 total flowers = 75
- White: 25% of 100 total flowers = 25
Now, apply the chi-square formula:
\[ \chi^2 = \sum \frac{(O - E)^2}{E} \]
Where \(O\) is the observed count and \(E\) is the expected count.
Calculating:
- For purple: \(\frac{(75 – 75)^2}{75} = 0\)
- For white: \(\frac{(25 – 25)^2}{25} = 0\)
So, \(\chi^2 = 0 + 0 = 0\).
Interpreting Results: The next step is interpreting the chi-square value. With a calculated \(\chi^2\) of 0 and degrees of freedom (df) of 1 (since we have two categories, we use df = n – 1), we can compare this against a chi-square distribution table.
At a significance level of 0.05, the critical value for df = 1 is 3.84. Since 0 < 3.84, we fail to reject the null hypothesis. This means our observed flower color distribution aligns with the expected Mendelian ratio. In simpler terms, our experiment supports Mendel’s findings!
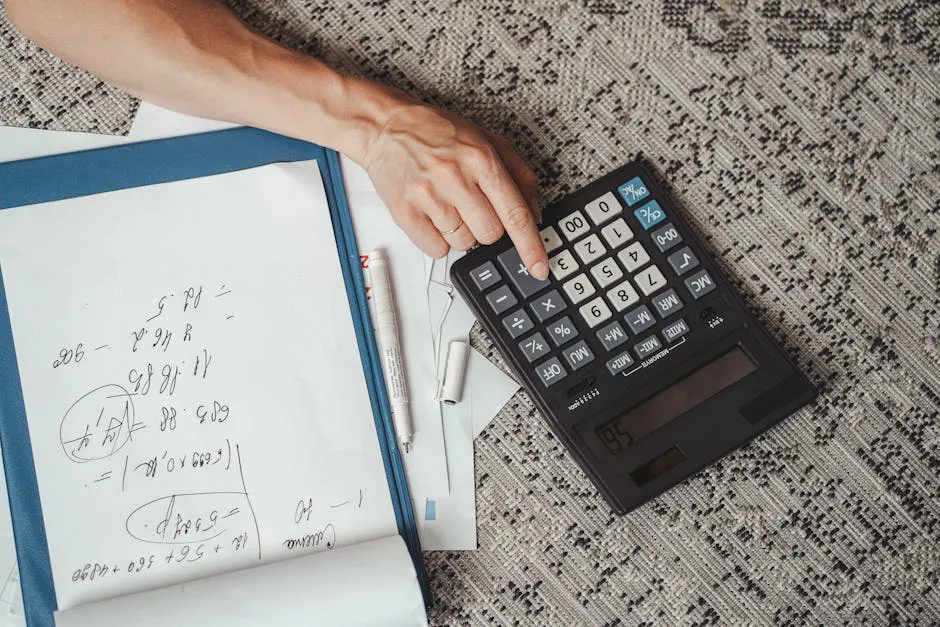
Real-World Applications of Genetics
Genetic Counseling
Understanding Mendelian genetics is crucial in genetic counseling. Parents with a family history of genetic disorders, like cystic fibrosis, seek guidance on the likelihood of passing on conditions to their children. By constructing pedigrees and applying probability, counselors can assess risks accurately. This allows families to make informed decisions about family planning and management of potential health issues.
Moreover, knowledge of inheritance patterns equips genetic counselors to provide tailored advice. If one parent is a carrier of a recessive trait, such as sickle cell anemia, understanding the probabilities aids in discussing the chances of having affected offspring. This knowledge empowers families, offering insight into their genetic landscape and informing reproductive choices.

Medical Implications
Genetic analysis isn’t just for family planning; it plays a pivotal role in diagnosing and managing inherited diseases. Take sickle cell disease, for example. By understanding the genetics behind the condition, healthcare professionals can implement early screening programs. This enables timely intervention for affected individuals, potentially improving their quality of life.
In the case of genetically linked cancers, such as BRCA mutations, awareness allows for proactive measures. Individuals with a family history can undergo genetic testing. Here, probability and pedigree analysis come into play, guiding them in making informed decisions about preventive surgeries or increased surveillance.
Additionally, genetic insights contribute to personalized medicine. Treatments tailored to an individual’s genetic makeup are becoming more common. This approach maximizes effectiveness and minimizes adverse effects. As research advances, we can expect genetic information to continue shaping the future of medicine, making it more precise and effective.
Conclusion
Recap
In this exploration of Mendelian genetics, we’ve unraveled the principles of inheritance, the roles of probability and pedigrees, and the significance of chi-square statistics. These concepts are not just theoretical; they hold real-world implications in genetic counseling and disease management.
Future Directions
Ongoing research in genetics promises to expand our understanding of complex traits and genetic disorders. As technology advances, the integration of statistical methods into genetic studies will enhance our ability to decipher intricate inheritance patterns. This opens up exciting possibilities for new discoveries and innovations in healthcare.
Call to Action
Want to dive deeper into the world of genetics? Explore resources available online, attend workshops, or even consider genetic counseling. Understanding your genetic makeup can empower you in making informed health decisions, impacting your life and future generations. Embrace the journey of discovery in genetics and its vast applications!
FAQs
Please let us know what you think about our content by leaving a comment down below!
Thank you for reading till here 🙂
All images from Pexels
First, calculate the expected numbers:
- Purple: 75% of 100 total flowers = 75
- White: 25% of 100 total flowers = 25
Now, apply the chi-square formula:
\[ \chi^2 = \sum \frac{(O - E)^2}{E} \]
Where \(O\) is the observed count and \(E\) is the expected count.
Calculating:
- For purple: \(\frac{(75 – 75)^2}{75} = 0\)
- For white: \(\frac{(25 – 25)^2}{25} = 0\)
So, \(\chi^2 = 0 + 0 = 0\).
Interpreting Results: The next step is interpreting the chi-square value. With a calculated \(\chi^2\) of 0 and degrees of freedom (df) of 1 (since we have two categories, we use df = n – 1), we can compare this against a chi-square distribution table.
At a significance level of 0.05, the critical value for df = 1 is 3.84. Since 0 < 3.84, we fail to reject the null hypothesis. This means our observed flower color distribution aligns with the expected Mendelian ratio. In simpler terms, our experiment supports Mendel’s findings!
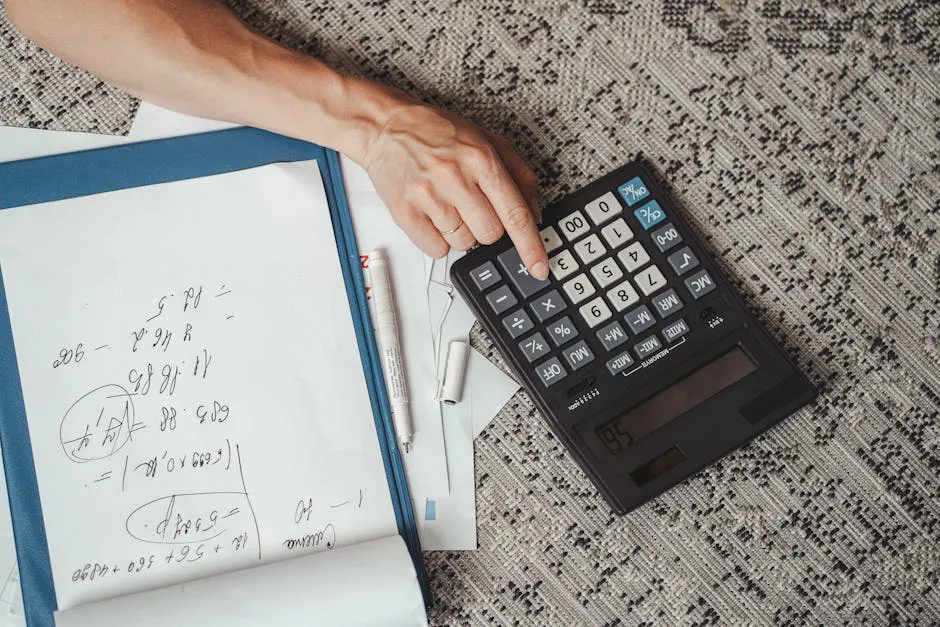
Real-World Applications of Genetics
Genetic Counseling
Understanding Mendelian genetics is crucial in genetic counseling. Parents with a family history of genetic disorders, like cystic fibrosis, seek guidance on the likelihood of passing on conditions to their children. By constructing pedigrees and applying probability, counselors can assess risks accurately. This allows families to make informed decisions about family planning and management of potential health issues.
Moreover, knowledge of inheritance patterns equips genetic counselors to provide tailored advice. If one parent is a carrier of a recessive trait, such as sickle cell anemia, understanding the probabilities aids in discussing the chances of having affected offspring. This knowledge empowers families, offering insight into their genetic landscape and informing reproductive choices.

Medical Implications
Genetic analysis isn’t just for family planning; it plays a pivotal role in diagnosing and managing inherited diseases. Take sickle cell disease, for example. By understanding the genetics behind the condition, healthcare professionals can implement early screening programs. This enables timely intervention for affected individuals, potentially improving their quality of life.
In the case of genetically linked cancers, such as BRCA mutations, awareness allows for proactive measures. Individuals with a family history can undergo genetic testing. Here, probability and pedigree analysis come into play, guiding them in making informed decisions about preventive surgeries or increased surveillance.
Additionally, genetic insights contribute to personalized medicine. Treatments tailored to an individual’s genetic makeup are becoming more common. This approach maximizes effectiveness and minimizes adverse effects. As research advances, we can expect genetic information to continue shaping the future of medicine, making it more precise and effective.
Conclusion
Recap
In this exploration of Mendelian genetics, we’ve unraveled the principles of inheritance, the roles of probability and pedigrees, and the significance of chi-square statistics. These concepts are not just theoretical; they hold real-world implications in genetic counseling and disease management.
Future Directions
Ongoing research in genetics promises to expand our understanding of complex traits and genetic disorders. As technology advances, the integration of statistical methods into genetic studies will enhance our ability to decipher intricate inheritance patterns. This opens up exciting possibilities for new discoveries and innovations in healthcare.
Call to Action
Want to dive deeper into the world of genetics? Explore resources available online, attend workshops, or even consider genetic counseling. Understanding your genetic makeup can empower you in making informed health decisions, impacting your life and future generations. Embrace the journey of discovery in genetics and its vast applications!
FAQs
Please let us know what you think about our content by leaving a comment down below!
Thank you for reading till here 🙂
All images from Pexels